Ocean Acidification: The SEA CO2 Filter Absorption of Dissolved Carbon Dioxide and its Production of Carbonates For Shell Building
- Wen Ying Ying Li
- Nov 13, 2024
- 19 min read
Updated: Nov 17, 2024
Wen Ying Ying Li, High School
Abstract
This paper centers around the question:“How Does Nature Capture Dissolved Carbon Dioxide In The Ocean and Utilize It For Intentional Functions?” and explores a biomimetic solution to mitigate ocean acidification, a pressing consequence of rising carbon dioxide emissions. The SEACO2 filter, inspired by the natural mechanisms of sea turtles and giant larvaceans, is designed to capture dissolved CO₂ in seawater and convert it into calcium carbonate, addressing the reduction of available carbonates critical for marine calcifying organisms. The filter mimics the larvacean’s intricate mucus house filtration system and integrates the sea turtle's biological process of releasing carbonates to neutralize acidity. Through a combination of solar-powered water turbines and innovative graphene-based filters, the SEACO2 filter absorbs carbon dioxide and initiates a chemical reaction with nickel to produce calcium carbonate. This byproduct is then released into the ocean, supporting marine life and mitigating acidification effects. Extensive design considerations, including material selection and energy sourcing, have been made to ensure the filter’s efficiency and sustainability. This project represents a potential breakthrough in oceanic carbon capture, merging biological principles with cutting-edge engineering to restore balance to marine ecosystems.
1. Background Information
According to the National Oceanic and Atmospheric Administration, 30% of the carbon emissions we release are absorbed into the ocean. Multiply that by 414.72 parts per million of atmospheric carbon dioxide, this leads us to approximately 124.42 parts per million of carbon dioxide absorbed into our oceans on a daily basis. When carbon dioxide is absorbed into the seawater, a series of chemical reactions create an increased concentration of hydrogen ions. The influx of hydrogen ions lowers the pH of the seawater, and at the same time reduces the availability of carbonate ions. Without a sufficient amount of carbonate ions, calcifying organisms such as oysters, clams, and sea urchins are particularly vulnerable because of their dependence on calcium carbonate for shell formation. When CO₂ from the atmosphere dissolves in seawater, it forms carbonic acid, which lowers the pH of the ocean. This reduces the amount of carbonate ions that these shellfish need to create their shells. As a result, their shells become weaker and thinner, making it harder for them to grow and survive.
Additionally, these shellfish need to use more energy to cope with the acidified conditions, which takes away from their growth and reproduction. If these shellfish populations decline, it can significantly affect the marine ecosystem. Shellfish provide important habitats and food for other marine animals. Without them, these habitats can break down, leading to less shelter and food for other species. This disruption can create more disorder, or entropy, in the ecosystem. The food web becomes less stable, and the overall health and balance of the marine environment will be compromised, impacting many other marine creatures that depend on shellfish. This ripple effect across the entire food chain thus could eventually put our primary source of protein at risk.
The influx of hydrogen ions that lower the pH of the water, and its consequent effects on shellfish is a phenomenon known as ocean acidification. It is one of the major consequences of what climate change has caused, and it is known to be a global threat to the world’s oceans, estuaries, and waterways. It has impacted not only our economy and vulnerable communities, but also ecosystems, food chains, and precious calcifying organisms that are now on the brink of extinction. Yet with the ceaseless human activity of burning fossil fuels, carbon dioxide emissions have continued to build up exponentially, trapping more carbon dioxide emissions into the ocean than scientists have ever seen since the start of the industrial revolution.
Figure 1. Atmospheric carbon dioxide graph from 1960-2021
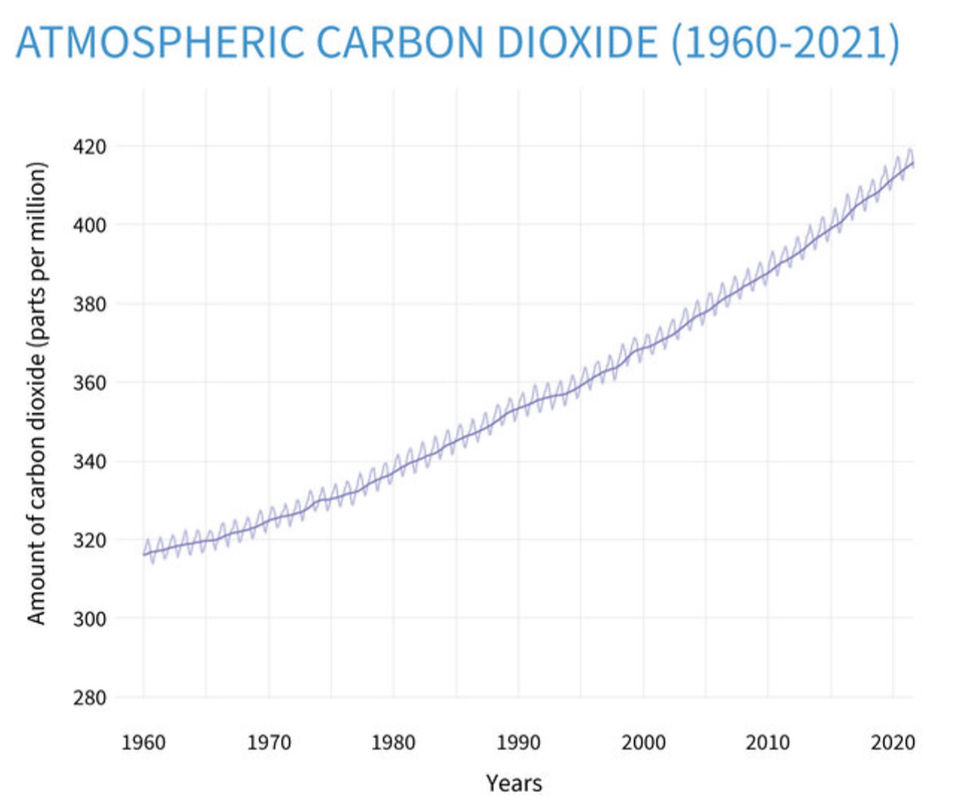
Although there have been large climate regimes such as the Paris Agreement, many times the criteria are so vague that it isn’t applicable to address ocean acidification. For example, although the Paris Agreement has set a target of limiting average global warming, it did not set a goal for limiting ocean pH change. Furthermore, in most cases, proposed design solutions utilize non sustainable materials, and the way the infrastructure is built may be harmful to marine life.
1.1. Theoretical Background
Carbon emissions are a major problem society faces today, because they contribute to greenhouse emissions leading to global warming and climate change. As a result, it catalyzes several negative impacts on various aspects of the environment, such as melting ice caps, disrupted ecosystems, and air pollution. Yet, despite the urgency of rising carbon emissions, many people neglect its consequences to our economy, welfare, and future. For example, it was recently reported by The Guardian that nearly 15% of Americans deny that climate change is real. On top of this, not many people are aware of the chemistry behind these environmental effects, thus they are unable to recognize that the root cause of these threatening issues are directly derived from our actions. An example like such would be ocean acidification.
Ocean acidification occurs when the pH (Potential of Hydrogen) level of the sea water lies within the lower left of the pH spectrum, ranging from 2 to 5. The normal pH of the ocean typically ranges from 8.15 to 8.2, and this alkaline degree is crucial for maintaining the health of marine ecosystems. However, due to increased carbon dioxide emissions, the pH of the oceans has been decreasing, leading to ocean acidification. Ever since the pre-industrial era, the average ocean pH has dropped to about 8.05, reflecting a roughly 30% increase in acidity. As we continue to burn fossil fuels, and release alarming amounts of carbon dioxide emissions into the atmosphere, it produces an excessive amount of carbonic acid. Whilst it is theoretically possible to slow down or even partially reverse this acidying process, doing so would require significant and sustained global efforts.
Carbonic acid is a weak acid that can be dissociated into hydrogen ions and bicarbonate ions. There are other chemical entities in the ocean too, such as calcium, magnesium, and carbonate ions, however, the excessive amounts of hydrogen ions produced disrupt the harmony between the chemicals in the ocean. This can particularly be seen as the number of available carbonate ions decrease due to the excess hydrogen ions chemically reacting with these available carbonate ions to form bicarbonate. Therefore there is a proportional relationship between burning more fossil fuels, and the formation of more bicarbonate in the ocean.
Without a sufficient amount of carbonate ions in the ocean, there will be less calcium carbonate produced which are the basic building blocks of shell building in calcifying organisms. As a result of this, shells and skeletons begin to deteriorate and eventually dissolve. Without the protection from shells, calcifying organisms, many of which define the bottom tier of the food chain, will die, disordering marine systems.
2. Identify
The first few stages of my biomimicry process revolved around analyzing the United Nations (UN) 17 Sustainable Development Goals (SDGs) that aimed to solve global issues in modern society today. From diminishing poverty to cultivating peace within worldwide institutions, I followed the path of my passions which lay upon SDG 13 - Climate Action. SDG 13 was selected for this paper because ocean acidification is a subsidy problem that is directly associated with the increasing carbon emissions that cause climate change. This SDG not only reflects a commitment to tackling an urgent critical issue of our time, but also demonstrates how aligning efforts together through engineering and design can allow us to create a more resilient and sustainable world.
To help us settle on a global issue, and garner more awareness on pressing problems around us, we first collectively discussed the causes, science, effects, and potential solutions to global warming in groups. An infographic of some notes discussed can be referenced as seen in the digital poster below. We learned of the various aspects of global warming such as rising temperatures, ocean acidification, and the loss of agriculture to name a few. Later on, with the Reframing Problems Activity, my partner and I investigated the disruptiveness of each issue and imagined ideal scenarios which we hope solutions would serve to achieve. The main theme we had in mind was to capture and remove carbon dioxide from the atmosphere. Delving into more research, we felt that carbon dioxide’s impact on oceans is an area comparatively neglected to its impacts on land. Thus this propelled us to center our design and paper on ocean acidification—a critical issue that not only threatens the health of the oceans, the livelihoods of people who depend on them, but also the overall stability of the Earth’s climate system.
Therefore with the help of the Youth Design Challenge, and brainstorming about what a successive Design Thinking Process looks like, the inspiration that we drew from both was a critical guide to the questions I would eventually create, and a sequence that sums up the ‘identify’ aspect of my biomimicry Design Thinking Process.
Figure 2. SDG 13 Infographic Figure 3. Reframing Problems Activity
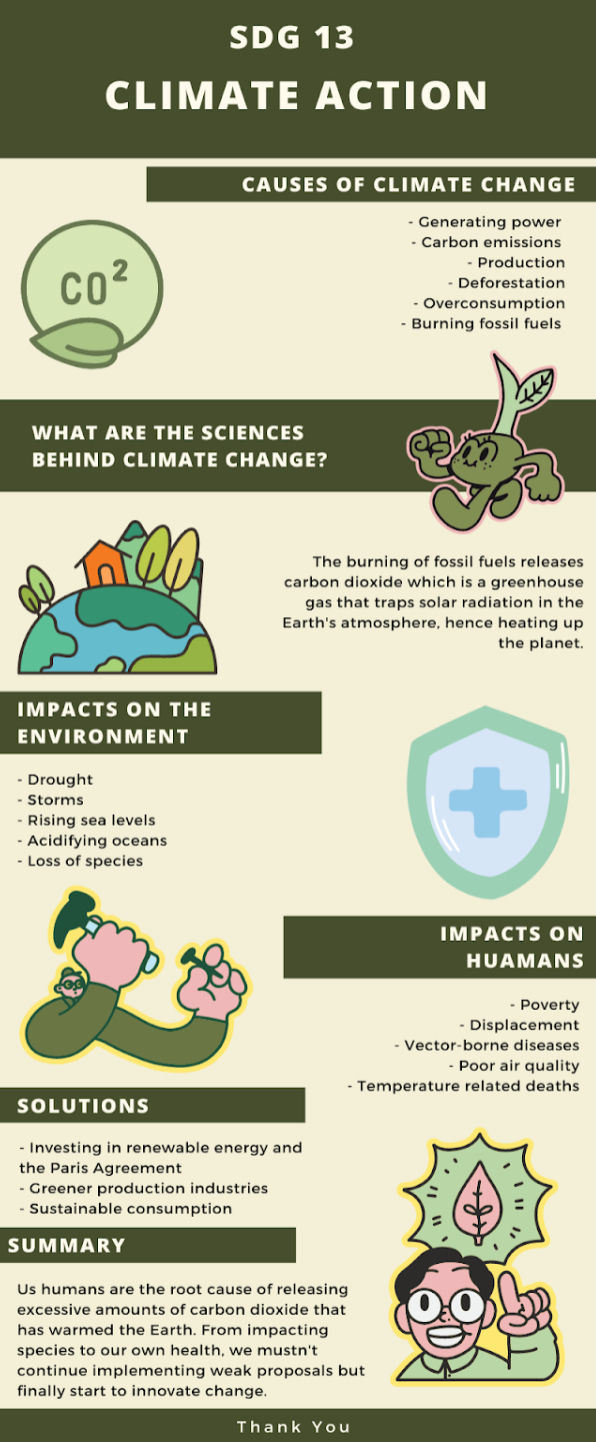
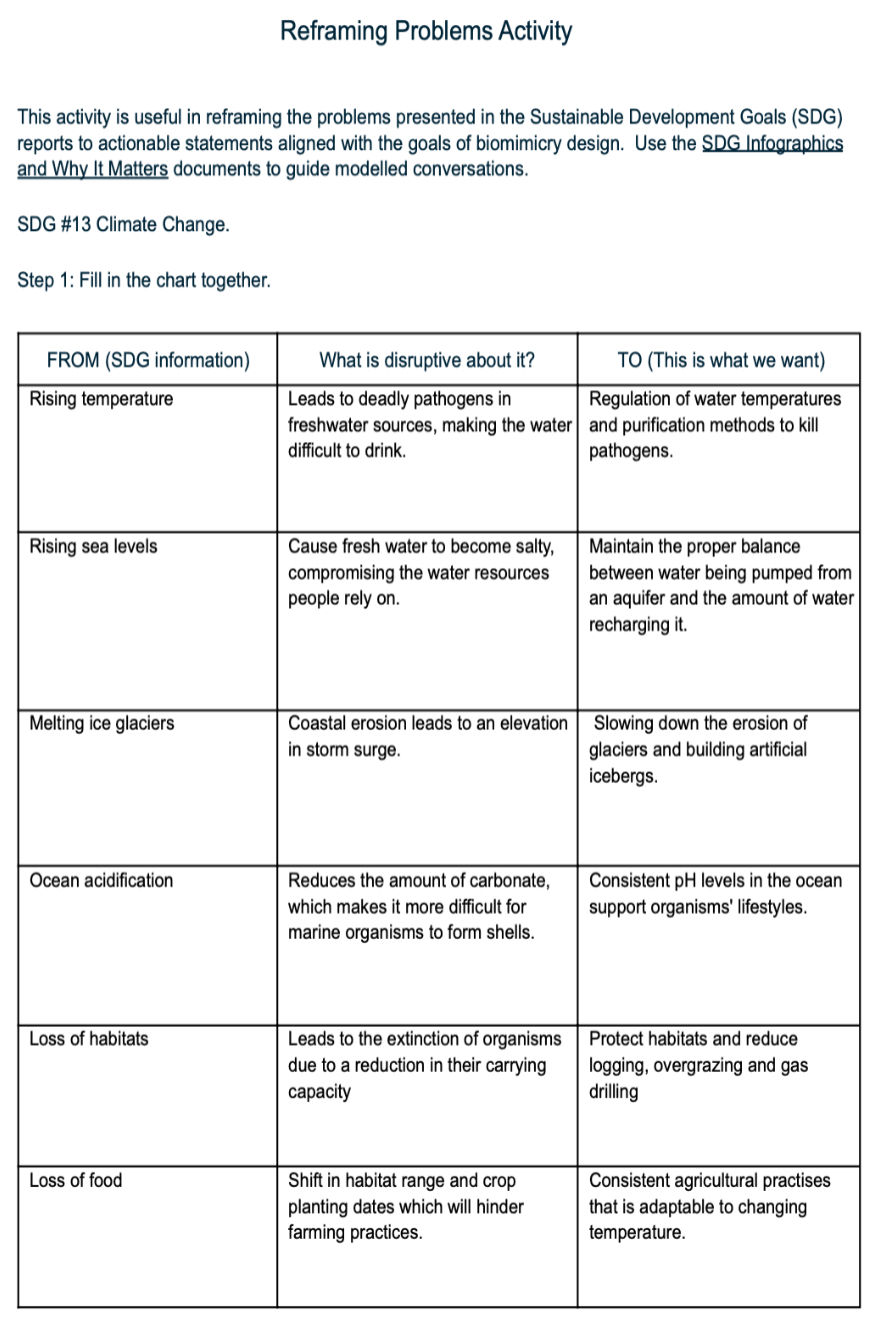
3. Explore
As my partner and I also hoped to incorporate SDG 14 - Life Below Water into our project, we began to narrow down our focus to problems that correlated both climate change and water bodies such as the ocean. Topics we identified interests in were; rising sea levels, fish migration, ocean acidification, coral bleaching, and marine heat waves. Alongside this information, we gathered extensive research by exploring various design problems within SGD 13 and understanding the functions, patterns, and solutions nature provides with the help of the AskNature website.
3.1. My Questions
We came up with a list of “How Might We…?” design questions in the field of our SGDs and explored a variety of ways in which we could tackle a problem by approaching the design questions differently. From this process, I not only learned about the criteria that help articulate the best-fit design question, but I also understood the importance of finding a balance between assumption and nuance. After a while of consideration, my partner and I eventually settled on the design question: “How Might We Capture Dissolved Carbon Dioxide In the Ocean?”
Figure 4. List of How Might We Design Questions
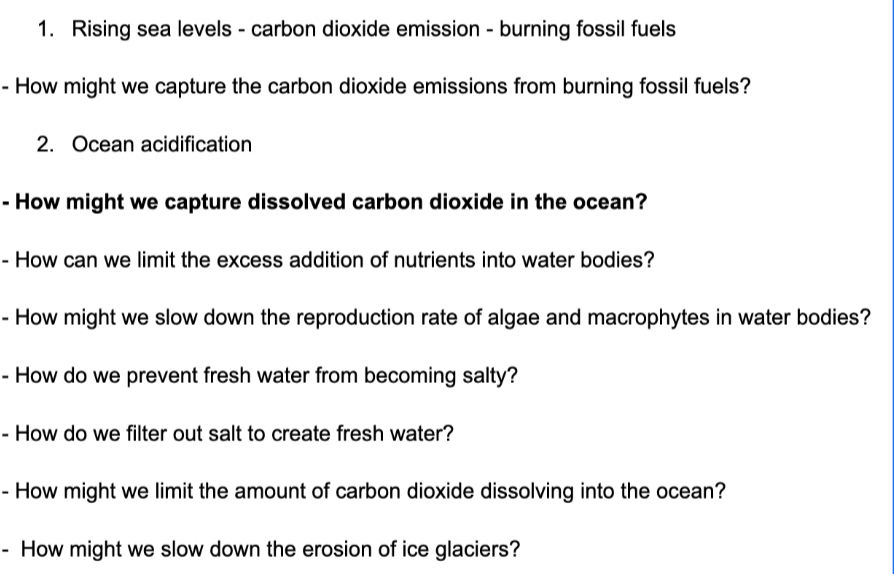
3.1.1 Biologize My Question
Now nearing the stage of creating, we transition our humanitarian focus on the design question, to an environmental one, by biologizing our project inquiry. The aid of listing down the functions and context revolving around our design question and potential solution, helped us explore the various approaches in which we could biologize our question.
Figure 5. Table to Biologize Design Questions
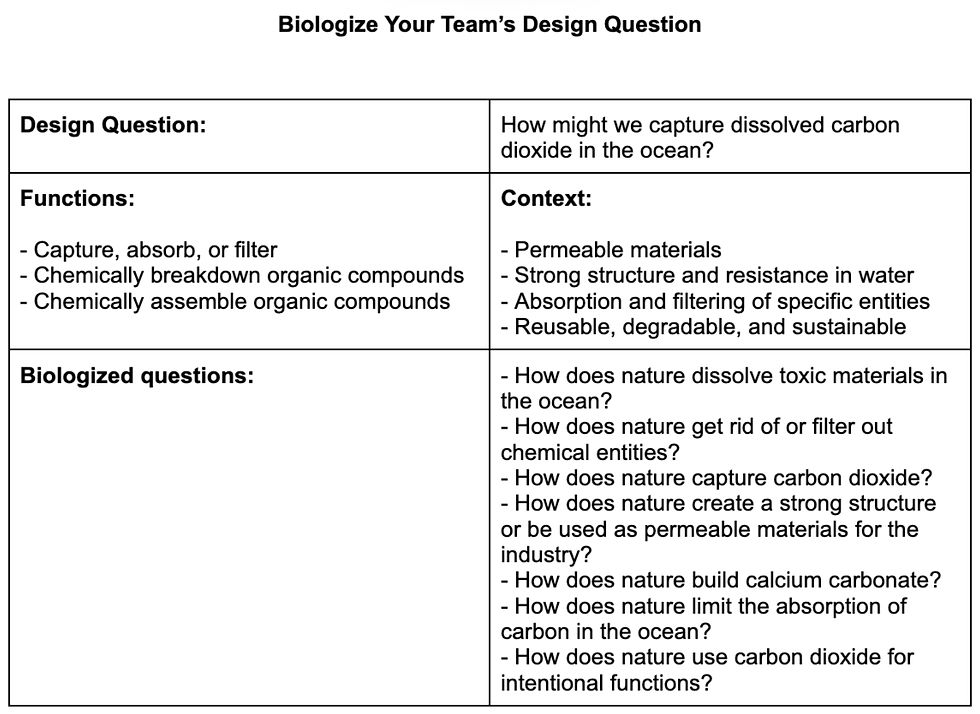
3.1.2 How Does Nature Do That?
What played a significant role in refining our biologized design question was utilizing the starting statement “How Does Nature…” As our final goal is to emulate nature’s characteristics in a proposed design solution, the “How Does Nature” starting statement was key in motivating us to consider how we could biologize our design question to emulate nature’s systems and patterns. By crafting a biologized question that can guide us to simulate nature in industrial designs, this made it easier for us to further our research in the next steps of our exploration, as we look into natural models such as organisms and ecosystems which can address the same function as our original design question.
3.2. Inspiration - Biological Strategy
We drew inspiration for our design by analyzing the biological strategies found in nature. With the help of the AskNature website, as well as the Developing Strategies Chart, I was able to emulate biological strategies and transform them into design strategies by highlighting keywords or phrases that are common in the function that my design will serve. This can be seen in the table below:
Figure 6. Developing Design Strategies Chart
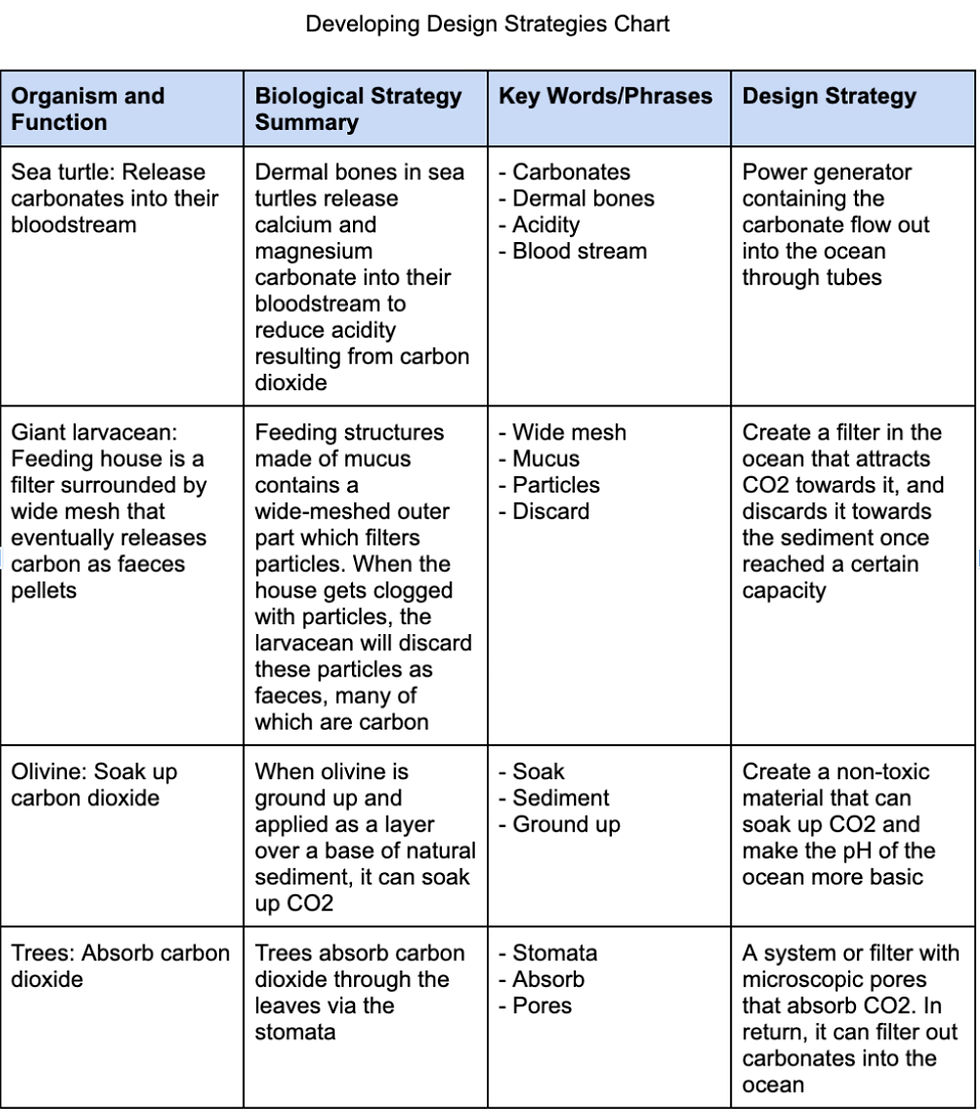
3.3. Brainstorm - Biological Strategy → Design Strategy
The biological strategy I chose to elaborate on for my design strategy is from the sea turtle and giant larvacean. Even though there are various solutions that we could draw from other organisms, I was fascinated by the filtering system of the larvacean’s mucus house and the ability of sea turtles to release carbonates.
Through research from MIT News and AskNature, I learned that giant larvaceans such as zooplankton have feeding structures built in the form of a complex filter that strains seawater for their tiny food. These complex filters are called “houses” and they are made of mucus that extends far past the body. The wide-meshed outer parts of the filter keep out big particles, and a smaller inner filter funnels food particles to the mouth via a tube. Eventually, when the giant larvacean's house gets clogged with particles, the animal discards these particles as feces and that’s when the species' contribution to carbon sequestration becomes apparent.
Due to being an organic structure, the houses are full of nutrients such as carbon. When abandoned, they sink to the bottom of the sea, where their carbon can stay sequestered, or excluded from going back into the atmosphere. Given the heavy weight of the larvacean filters, the carbon sinks more rapidly than smaller organic particles, reducing the time taken to degrade in the upper ocean where the carbon would enter the atmosphere. Although many would assume that this biological filter is powered by the internal energy of the organism, interestingly, the filter is rather powered by the consistent motion of the animal’s tail, which directs water inside and through the house.
On the other hand, the biological strategy for sea turtles is their ability to release calcium and magnesium carbonates into their bloodstream from their dermal bones in high acidity. When CO₂ levels in the blood increase, it forms carbonic acid, which dissociates into hydrogen ions and bicarbonate, leading to a decrease in blood pH (acidosis). To counter this, sea turtles will release calcium and magnesium carbonates from their bones. These carbonates act as buffers by reacting with the excess hydrogen ions, thereby helping to neutralize the acidity and stabilize blood pH. This process is part of a broader physiological response that helps marine reptiles like sea turtles maintain homeostasis in varying environmental conditions, particularly when diving, where CO₂ levels can rise due to limited gas exchange.
Overall, I enjoyed learning about these biological strategies that are interconnected with the same function. I saw parallels between these two features and hence planned to combine both to create a potential design.
4. Create
4.1. Brainstorm Solutions - Sketching
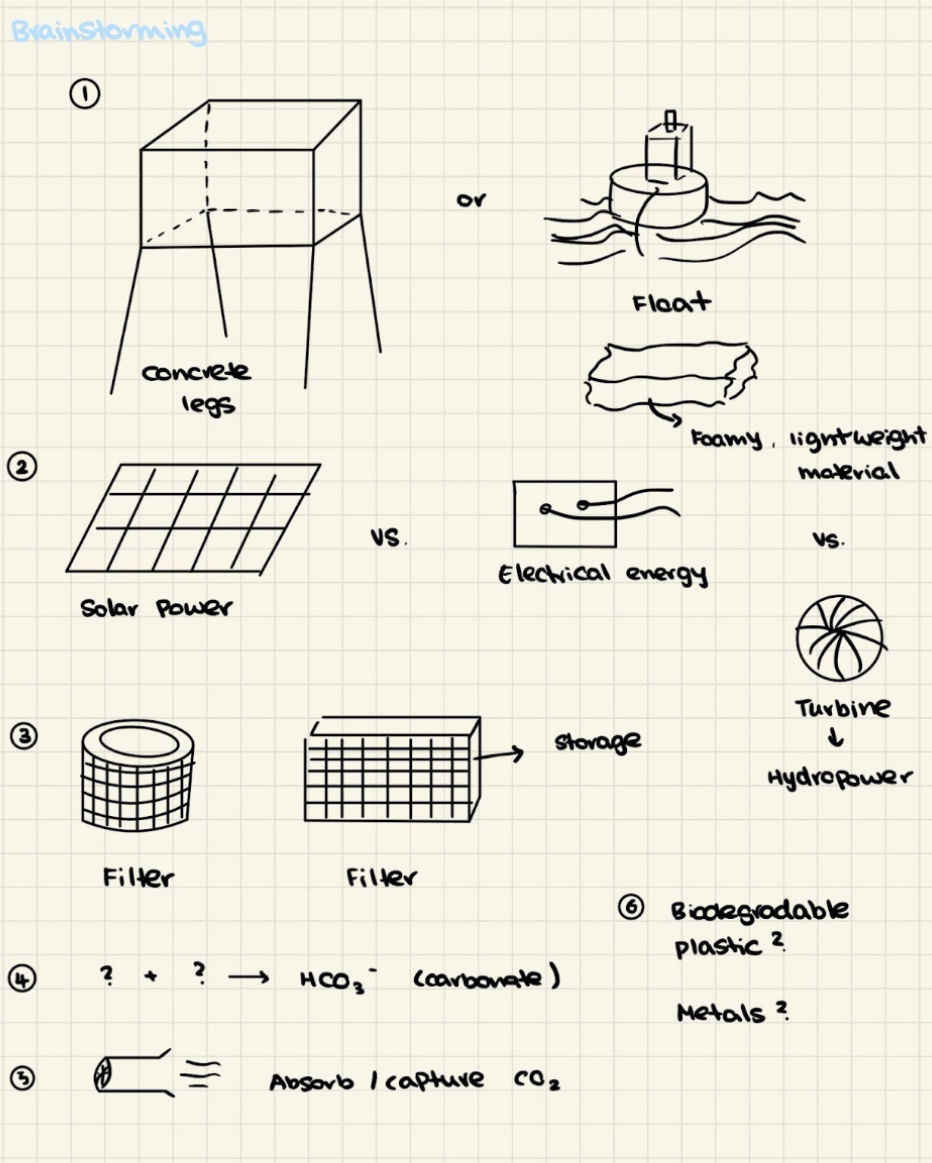
Figure 7. Design Brainstorming Sketches
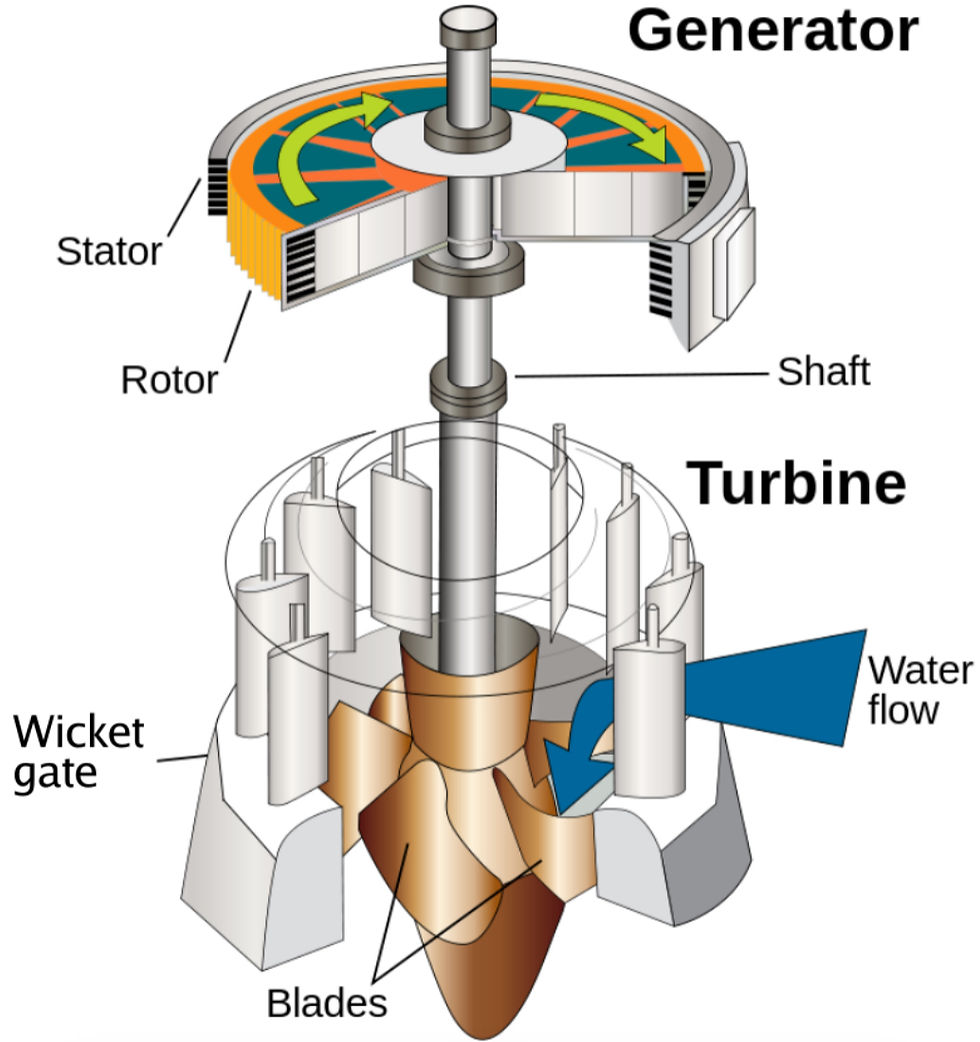
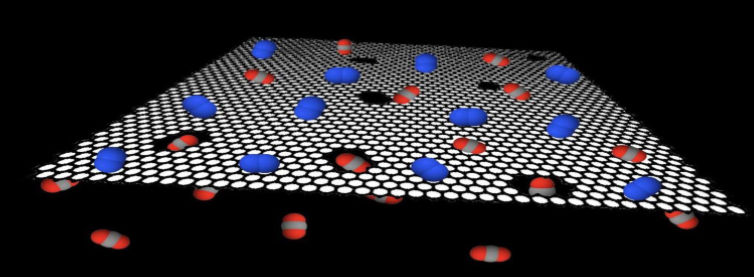
Figure 8. Three-phase AC Synchronous Generator Figure 9. Graphene Carbon Dioxide Filter
These are parts of the potential design which I brainstormed. I weighed the pros and cons of utilizing different types of materials, ways to source energy, and how the design could function to target its main goal. This played a critical role in exploring various ideas, and methods to approach the same function from different perspectives. As I wanted to mimic the motion of the mucus tail as a source of energy to direct water inside and through the house, I chose to incorporate a water turbine instead of using electrical energy with wires. Additionally, the giant larvacean's complex feeding structure - the mucus house inspired our incorporation of two filters in the design. Mirroring the wide-meshed outer parts of the filter that keep out big particles, and a smaller inner filter to funnel food particles to the mouth via a tube, each of the filters in my design will have a specific layer for capturing and a consequent storage area. Furthermore, to emulate the sea turtle's biological strategy of releasing carbonates into their bloodstream, I decided to design a tank in the infrastructure that stores the catalyst Nickel, so that the two reactants can react and produce the product calcium carbonate. This will then be released into the seawater from a latch.
4.2. My Design Solution
My design - the SEACO2 filter targets the major effect of ocean acidification, which is the insufficient amount of carbonate available to bond with calcium ions to form calcium carbonate which is the basic building block to build shells.
It functions to do so by absorbing seawater with the help of solar panels and water turbines. Although at the start of my brainstorming process, I considered including only one water turbine. However, after pondering on the energy usage and the context of the design, I decided to incorporate two water turbines so that there will be more power to vacuum in the seawater. In addition to the water turbines, there will be a large solar panel on top of a thick layer of biodegradable foam so that not only will there be energy to source the water turbines, but the design will also be able to float on the surface of the ocean. Furthermore, the solar panel and thick layer of biodegradable foam are detachable so that employed staff would be able to access the storage of catalyst Nickel so that the chemical entity could be continuously restored.
My design will also include two filters. Even though the main purpose is to filter out carbon dioxide, due to a concerning amount of microplastics and ocean waste in the seawater, there will be a filter incorporated to filter out waste and proceed to transport them into storage at the back of the machine. After filtering as well as storing all of the microplastics, seawater will move towards the second filter where carbon dioxide is captured. The CO₂ will be absorbed into an area where there is storage at the top. The storage contains the catalyst Nickel. When the filter containing the captured CO₂reaches a specific capacity, the latch to the above storage will open, releasing the Nickel into the store of carbon dioxide, to cause a reaction. This reaction will consequently result in the production of calcium carbonate. Now with more calcium carbonate in the ocean and a continuous absorption of carbon dioxide, I hope that this design will achieve harmony between these chemical entities in the ocean, and reverse the disrupted system of marine life and ocean acidification.
Materials are very critical in the solution stage. Although I researched various methods to manufacture the same design, I settled on layering the exterior of the design with nanotechnology, having the box be made out of metal, and the floating device made out of biodegradable foam.
In terms of the filter, as it was ideal for it to be permeable, accurate and light, I thought of using a chemical compound known as graphene which is a one-atom-thick layer of carbon atoms arranged in a hexagonal lattice. Graphene is very strong due to its covalent bonds, and is also the thinnest material possible, as it is only one atom thick. With inspiration from the Swiss Federal Institute of Technology Lausanne, I replicated their graphene filters into the filters in my design to isolate microplastics and capture carbon dioxide. According to Professor Kumar Varoon Agrawal at EPFL’s School of Basic Sciences, he says, “Our approach was simple. We made carbon dioxide-sized holes in graphene, which allowed carbon dioxide to flow through while blocking other gasses such as nitrogen, which are larger than carbon dioxide.”
Henceforth, the filters to capture carbon dioxide though will be built of graphene, the molecular holes will be built according to the shape of carbon dioxide molecules. However, I would say that much progress and complex research would have to be spent exploring the materials, as one slight change could result in a complex disruption in the workings of the filter. One type of filter material I am interested in exploring more is single-walled carbon nanotubes. 4.2. My Design Solution
My design - the SEACO2 filter targets the major effect of ocean acidification, which is the insufficient amount of carbonate available to bond with calcium ions to form calcium carbonate which is the basic building block to build shells.
It functions to do so by absorbing seawater with the help of solar panels and water turbines. Although at the start of my brainstorming process, I considered including only one water turbine. However, after pondering on the energy usage and the context of the design, I decided to incorporate two water turbines so that there will be more power to vacuum in the seawater. In addition to the water turbines, there will be a large solar panel on top of a thick layer of biodegradable foam so that not only will there be energy to source the water turbines, but the design will also be able to float on the surface of the ocean. Furthermore, the solar panel and thick layer of biodegradable foam are detachable so that employed staff would be able to access the storage of catalyst Nickel so that the chemical entity could be continuously restored.
My design will also include two filters. Even though the main purpose is to filter out carbon dioxide, due to a concerning amount of microplastics and ocean waste in the seawater, there will be a filter incorporated to filter out waste and proceed to transport them into storage at the back of the machine. After filtering as well as storing all of the microplastics, seawater will move towards the second filter where carbon dioxide is captured. The CO₂ will be absorbed into an area where there is storage at the top. The storage contains the catalyst Nickel. When the filter containing the captured CO₂reaches a specific capacity, the latch to the above storage will open, releasing the Nickel into the store of carbon dioxide, to cause a reaction. This reaction will consequently result in the production of calcium carbonate. Now with more calcium carbonate in the ocean and a continuous absorption of carbon dioxide, I hope that this design will achieve harmony between these chemical entities in the ocean, and reverse the disrupted system of marine life and ocean acidification.
Materials are very critical in the solution stage. Although I researched various methods to manufacture the same design, I settled on layering the exterior of the design with nanotechnology, having the box be made out of metal, and the floating device made out of biodegradable foam.
In terms of the filter, as it was ideal for it to be permeable, accurate and light, I thought of using a chemical compound known as graphene which is a one-atom-thick layer of carbon atoms arranged in a hexagonal lattice. Graphene is very strong due to its covalent bonds, and is also the thinnest material possible, as it is only one atom thick. With inspiration from the Swiss Federal Institute of Technology Lausanne, I replicated their graphene filters into the filters in my design to isolate microplastics and capture carbon dioxide. According to Professor Kumar Varoon Agrawal at EPFL’s School of Basic Sciences, he says, “Our approach was simple. We made carbon dioxide-sized holes in graphene, which allowed carbon dioxide to flow through while blocking other gasses such as nitrogen, which are larger than carbon dioxide.”
Henceforth, the filters to capture carbon dioxide though will be built of graphene, the molecular holes will be built according to the shape of carbon dioxide molecules. However, I would say that much progress and complex research would have to be spent exploring the materials, as one slight change could result in a complex disruption in the workings of the filter. One type of filter material I am interested in exploring more is single-walled carbon nanotubes.
Figure 10. Design Solution Sketch
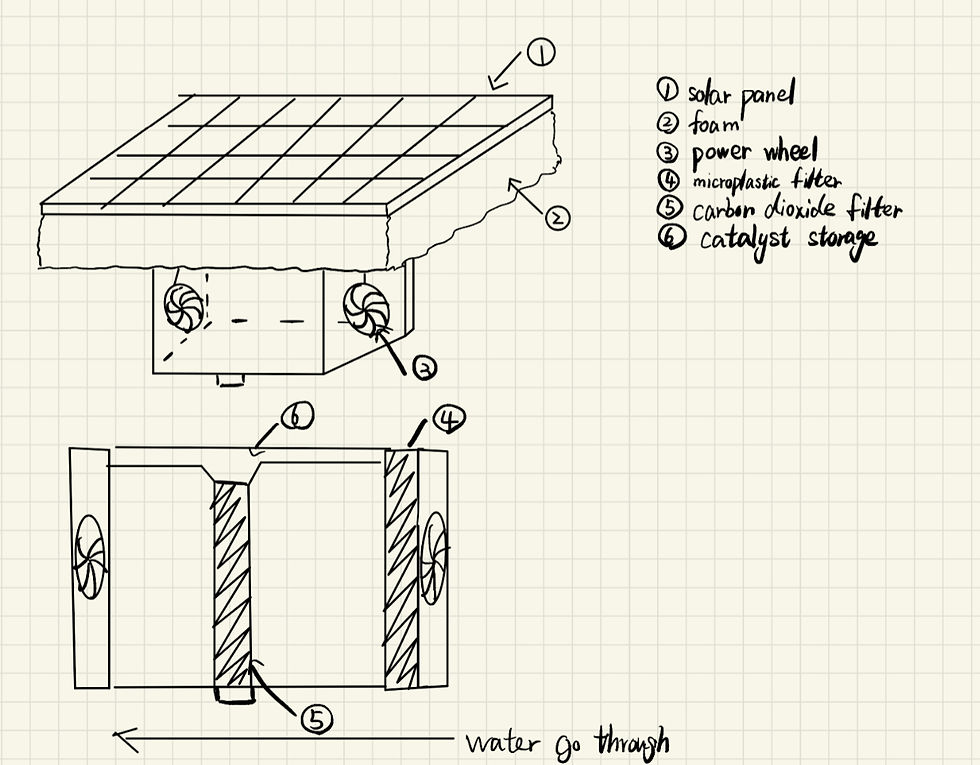
5. Refine
Figure 11. CAD Design Close-up Figure 12. CAD Design Wide-shot
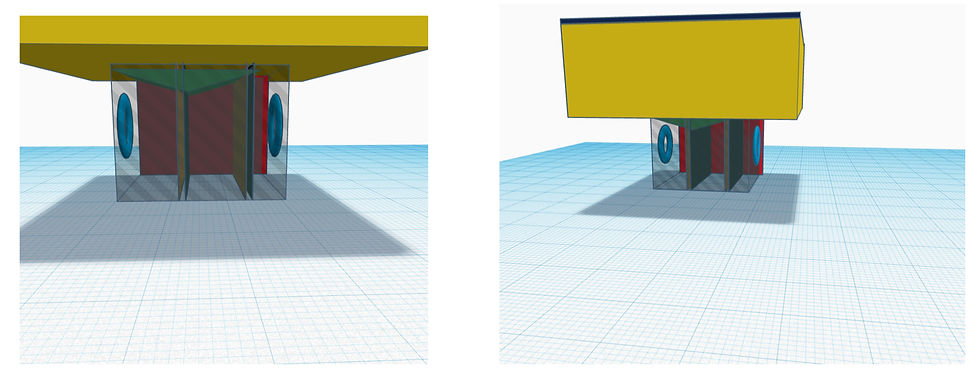
After several stages of refining and adjusting, my partner and I created a CAD model of our final design product on Tinkercad. The diagram illustrates how the design would look on a 3D scale, and provides insight into how the interior of the metal box would look, showcasing the two filters for microplastics and carbon dioxide respectively. Furthermore, the dark blue box on the surface is the solar panel, and the thick yellow rectangle below is the foam for floating. The red storage is where the microplastics would be kept, and the green top is the storage of catalyst Nickel, where a latch would release the Nickel and react with filtered carbon dioxide to produce calcium carbonate. Additionally, the two blue circles on the side depict the water turbines that will vacuum seawater through hydropower.
6. Evaluate
6.1. Constraints
Limitations to our design include the location and space for where the design will be placed, how and who can renew the machine, how the data can be monitored, as well as how we can guarantee that the carbonate produced will not create any adverse effects. The location and space for placement are critical, as the filter must be positioned in areas with high CO₂ concentrations or severe acidification without disrupting sensitive ecosystems. Conducting environmental impact assessments and involving local stakeholders can help select appropriate sites, while modular designs can adapt to spatial constraints. Additionally, maintenance and renewal are also essential; however, the specialized knowledge required may limit who can perform these tasks. To address this, developing an automated system for basic maintenance and partnering with local personnel or marine institutions for more complex upkeep could be a potential route to consider. Data monitoring, on the other hand, presents another challenge due to the harsh marine environment, but using robust sensors and cloud-based platforms could ensure reliable data collection and real-time analysis. Finally, the production of carbonate by the filter must be carefully managed to prevent adverse effects on marine life. By conducting thorough environmental testing and monitoring the filter’s impact, carbonate can be released in controlled, safe amounts, ensuring that the filter effectively mitigates ocean acidification without causing unintended harm.Therefore extensive testing alongside a team of researchers and engineers is essential to revitalize this design.
Indications of failure would be the latch to the carbonate not opening properly, for it to be released into the seawater. The catalyst Nickel and carbon dioxide do not chemically react to form the compound calcium carbonate, and whether or not there will be any adverse effects created from the machine or chemicals towards marine life.
Furthermore, I’m curious as to how and where we would source the catalyst Nickel ethically, and whether this would bring good or more harm to the environment. Though it is yet to be explored, a sign of failure I’m interested to investigate is whether or not the catalyst Nickel has an expiration date, and how that will be put into consideration for when the solution is implemented in the ocean. Additionally, similar to the aging of sea turtles and giant larvaceans, will these organisms stop functioning the biological strategies when they age, and how would we see this parallax in industrial processes?
Moreover, since the design would indeed be adding a chemical into ocean water, this may spur societal concerns. Thus to implement this solution at a large scale in the ocean, it would be a long-term goal that requires extensive testing. A small tank simulation hence can provide valuable insights, but the margin of error would be significant when scaling up due to differences in water volume, flow dynamics, and ecosystem interactions. Additionally, to identify the most appropriate amount of Nickel to be released in the ocean without damaging species, Nickel concentration must be controlled carefully, as many marine species, including some fish, invertebrates, and algae, are sensitive to nickel toxicity. Alongside this, the simulation should also test the effects of the solution on varying environmental conditions to accurately predict the broader impact. Therefore to ensure that adding nickel externally maintains ecological balance requires precise dosing, continuous monitoring, and robust recovery systems to prevent accumulation and adverse effects.
Given the short duration of time, I’m not able to build a physical model or test the practicality of the solution, however, I really enjoyed the learning process and researching all the new information I’ve accumulated. Being able to immerse and experience the design engineering process from the root problem, all till extensive research, and continuous iterations really inspired me, allowing me to build on the essential skill of an engineer—patience. With the given knowledge I’ve learnt, I will spread awareness about this cause in school, and not only present my design to garner more attention on ocean acidification, but also to simply be more conscious of how my daily actions can greatly affect the environment.
7. References and Citations
Admin. (2020, October 16). Covering ocean acidification: Chemistry and considerations » Yale climate connections. Yale Climate Connections. https://yaleclimateconnections.org/2008/06/covering-ocean-acidification-chemistry-and-considerations/
AskNature. (n.d.). Innovation Inspired by Nature — AskNature. https://asknature.org/strategy/dermal-bone-buffers-co2-induced-acidosis/
An economical way to convert carbon dioxide into calcium carbonate. (2013, February 5). NextBigFuture.com. https://www.nextbigfuture.com/2013/02/an-economical-way-to-convert-carbon.html
Graphene: Structure and shape. (n.d.). Graphene Info | The Graphene Experts. https://www.graphene-info.com/graphene-structure-and-shape
Mehar, P. (2021, March 3). A graphene filter for efficient carbon capture. Tech Explorist. https://www.techexplorist.com/graphene-filter-efficient-carbon-capture/38096/
Mucus traps carbon from Seawater — Biological strategy — AskNature. (n.d.). Innovation Inspired by Nature — AskNature. https://asknature.org/strategy/mucus-filters-and-traps-carbon-from-seawater/
Putting carbon dioxide to good use. (n.d.). MIT News | Massachusetts Institute of Technology. https://news.mit.edu/2010/belcher-carbon-0922
Sea urchin inspires carbon capture catalyst. (2013, February 5). Chemistry World. https://www.chemistryworld.com/news/sea-urchin-inspires-carbon-capture-catalyst/5863.article
What is ocean acidification? (n.d.). NOAA Pacific Marine Environmental Laboratory (PMEL) |. https://www.pmel.noaa.gov/co2/story/What+is+Ocean+Acidification%3F
Atmospheric Carbon Dioxide Graph 1960-2021: Climate.gov. “ClimateDashboard-Atmospheric-Carbon-Dioxide-Graph-20230606-1400px.png | NOAA Climate.gov.” www.climate.gov, www.climate.gov/media/15284.
Image of Three-phase AC Synchronous Generator: HNAC Technology. “Three-Phase AC Synchronous Generator, China Three-Phase AC Synchronous Generator Manufacturers, Suppliers, Factory - HNAC Technology Co., Ltd.” www.hnacglobal.com/Generator112/generator.
Image of Graphene Carbon Dioxide Filter: Agrawal, Kumar. “World’s Thinnest Graphene Filter and Its Use in Carbon Capture.” Advances in Engineering, 24 Feb. 2021, advanceseng.com/worlds-thinnest-graphene-filter-carbon-capture/
コメント